The intricate science behind CNC tooling
John Parsons was born in Detroit in 1913. Thanks to Henry Ford and others like him, that was a good place to grow up if you wanted to be an…
John Parsons was born in Detroit in 1913. Thanks to Henry Ford and others like him, that was a good place to grow up if you wanted to be an inventor. After two world wars and an economic depression, Parsons was working at Wright-Patterson Air Force Base near Dayton, Ohio during the late 1940s. He and his assistant, Frank Stulen, were designing propeller blades for helicopters when Parsons conceived the notion of using nascent computer technology to control the path of a cutter.
The two men used their new process to make tapered, complex wings for military aircraft. Their partners included both engineers at IBM, who were developing the first commercially viable computers, and a group of researchers at MIT who were working on servomotors and the computer programs to control them. Those efforts eventually became G-code.
In 1958, Parsons received a patent that he had filed six years earlier for a “motor-controlled apparatus for positioning a machine tool.” From 1968 until his retirement 18 years later, he was the president of the John T. Parsons Co. in Traverse City, Mich., which designed and built parts for the aeronautics industry. He was awarded numerous academic and industry honors through the years and is sometimes referred to as the “Father of the Second Industrial Revolution.”
Parsons died in 2007 at the age of 93, having lived long enough to see his inventions revolutionize several industries including plastics, non-ferrous metals, foam and, of course, woodworking.
Tooling up
Woodshops can thank IBM, MIT, Parsons and Stulen for the platform, mechanics, motors and code that became CNC. But the tools that these machines move and control are a science all to themselves.
For a woodshop just getting into CNC machining, that means there’s a lot to learn. For example, the tools (usually router bits) can be one piece of steel with high tungsten content, so that a cutting profile that has been ground into the shaft will hold its edge. Or a tool might be made up of a steel shaft with carbide inserts that can be changed when dull.
Tools can usually be sharpened or re-tipped. They can also be custom made for a woodshop at remarkably affordable prices considering the complex technology involved. This lets a woodworker do custom tasks such as match a profile for a historic renovation or perhaps mill an unusual molding that has been specified by a hotel or restaurant chain.
Carbide is the most common hardener used in CNC tooling and woodworkers are generally familiar with its properties because they have been using over-the-counter bits made for portable routers and table saw blades with carbide tips. However, a little knowledge can be a dangerous thing and choosing a grade of carbide for CNC tooling based only on one’s experience with small, high-speed cutters is probably not the best idea. The choice is perhaps best left to an experienced tool supplier or designer. That’s because a woodshop that specifies a grade might be surprised by the end result.
Carbide is complex, both in its grading and its manufacture. When iron is being turned into steel, the mill can change the nature of the finished product by adding various other metals to control certain properties. When a secondary element is added, the steel becomes an alloy. Molybdenum is used to form the carbides that bring strength and endurance to CNC tooling. Carbide is a compound that contains carbon along with one or more elements that have a lesser electro-negative ability (these are bonding agents). When a woodshop manager asks a CNC tooling manufacturer to use something such as C2, C4 or some other grade of carbide, they are asking the indefinable.
“Cross-referencing carbide by grade is like comparing burgers from Wendy’s, Burger King and McDonald’s,” says Tom Walz of Carbide Processors in Tacoma, Wash. “There is no comprehensive comparison of tungsten carbide between and among tungsten carbide suppliers. A big part of the problem is the huge number of suppliers, grades and trade names. There are at least 5,000 different grades of tungsten carbide sold under more than 1,500 different trade names by more than 1,500 different companies.”
All that, of course, only adds to the confusion for a shop that has just purchased a brand-new CNC router and needs to know something about bits. The best advice is to speak with a reputable supplier (check out the resource guide on the Woodshop News website), and describe the task you need to perform including the material being worked, its thickness, a drawing of the profile/shape that needs to be milled and the lineal footage that will be routed. The supplier can then recommend solutions that meet your needs.
Other tooling choices
Stellite is a trademarked product belonging to Kennametal Co. of Latrobe, Pa. The company’s founder, metallurgist Philip McKenna, discovered the compound in 1938. It’s a tungsten-titanium carbide alloy specifically made for cutting tools. The alloy is used in a range of cobalt-chromium compounds that are designed for wear resistance. Stellite can also contain tungsten or molybdenum and a small measure of carbon.
Polycrystalline diamond (PCD) is a composite of diamond particles sintered together with a metallic binder. Not all CNC operators are sold on manmade diamonds as a tooling material. So if a tool is available in both carbide and diamond, it might be a good idea to test both on a long run and see which lasts the longest and which delivers the cleanest edges.
Chip load factor
Another way to measure performance and also to develop guidelines for the speed at which a CNC tool should be moved through the work is a concept called “chip load.” This is often supplied by a CNC tooling manufacturer right on the packaging and appears as a range of numbers such as “.024-.026’.”
Chip load describes the thickness of the chip that is being removed by each cutting edge of the tool. The idea is to adjust the speed at which the spindle is turning and the speed at which the tool is being moved, so that the cutting edges do their work most efficiently. That efficiency is a balance between how fast jobs are being completed and how fast the tooling becomes dull. Overheating is an issue and it can be caused when the tool is being asked to do too much work too quickly. But slowing everything down too much can also cause problems, including burn marks on the material being cut because the bit is staying in one place too long.
Chip load can be calculated fairly easily. Start by noting the feed rate the machine is currently set to: this measures how fast the tool is being moved in “inches per minute.” Then note the revolutions per minute at which the tool is being spun in the spindle. Count the number of flutes (cutting edges) on the bit and you’re ready to apply this formula:
Feed rate/(RPM x number of flutes) = Chip load
For example, if the tool is traveling at 500 inches a minute and the spindle is turning a three-flute bit at 10,000 rpm, the chip load would be 0.0166.
A tool with a diameter of 3/8” that is cutting a mid-range hardwood such as oak will probably want to have a chip load in the 0.015 to 0.018 range. For MDF, the ideal number might be closer to 0.021. To get there from our example, one might switch to a two-flute bit and up the RPMs to 12,000. Decreasing the feed rate and increasing the RPMs a little more might also deliver a better, burn-free cut.
Some experimenting is required because of variables. For example, not all MDF is made with the same grain and resin structure and not all tools are made with the same carbide structure. The manufacturer of the CNC router will have guidelines on choosing tooling and the speeds at which to work.
This article originally appeared in the April 2015 issue.
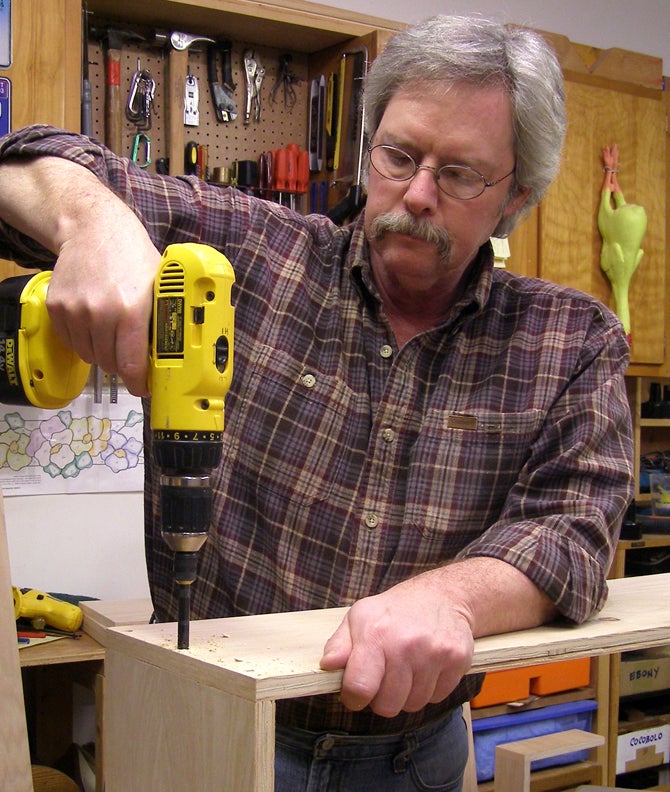
A.J. Hamler is the former editor of Woodshop News and Woodcraft Magazine. He's currently a freelance woodworking writer/editor, which is another way of stating self-employed. When he's not writing or in the shop, he enjoys science fiction, gourmet cooking and Civil War reenacting, but not at the same time.